8 mars 2022
Climate Change Initiative contributes to IPCC Working Group II report
New report assesses climate change impacts, vulnerability and adaptation
Earth observation information has made a significant contribution to the IPCC’s latest report [28 February] from Working Group II, focussed on climate change impacts, vulnerability and adaptation.
The new report assesses the disruption caused by human-induced climate change across nature and impacts to billions of lives around the world. The report’s authors call for ambitious, accelerated action to both adapt to climate change and cut in greenhouse gas emissions to avert worsening the situation.
Satellite contribute to IPCC Sixth Assessment report
Several studies and satellite-based datasets from ESA’s flagship Climate Change Initiative (CCI) are included and support the report’s headline statements. This brings the number of contributing citations from the CCI to almost 400 across the IPCC Sixth Assessment reports from Working groups I and II.
Generated from multiple satellite missions, the long-term datasets generated by the CCI provide the observation record for key aspects of the climate. Collectively they help understand the physical climate while and providing an important tool for technology-based adaptation solutions, including early-warning systems of extreme events, environmental monitoring, improved forecast and hindcast models.
Increasing extremes
While the Working Group I report details the physical state of the climate, the latest assessment warns of increasing frequency and severity of climate extremes – something that will worsen with rising global temperature. Satellite-based studies played a key role in supporting this key takeaway message, with research and datasets from the CCI revealing impacts across freshwater, ocean, coastal, mountain and polar systems.
New research using the relatively new Lakes_cci dataset, shows impacts across the world’s freshwater lakes, with lake surface temperatures increased. Used to support model projections, these observed data point to more severe lake heatwaves by the end of the century point as global temperature rises. The knock-on effects on freshwater ecosystems, including biodiversity and water quality are highlighted, with the changes likely to impact surrounding communities.
Rising temperatures are causing profound and extensive changes in the global cryosphere. Mountain glaciers, land ice and snow cover shrinking, causing substantial, permanent impacts on the ways of life of people in these regions, particularly Indigenous Peoples with strong cultural links to long-term or seasonally frozen environments. For example, there is a consistently negative snow-mass trend between 1981-2018 across north America, based on the CCI snow project’s dataset. The corresponding CCI glacier project, which maps glacier mass change on a global scale, is cited as evidence of the accelerating glacier mass loss over the observation record. As well as resulting falling freshwater availability impacting irrigation, hydropower production and tourism, the present rate of glacier melt is contributing to 25-30% of global sea level rise.
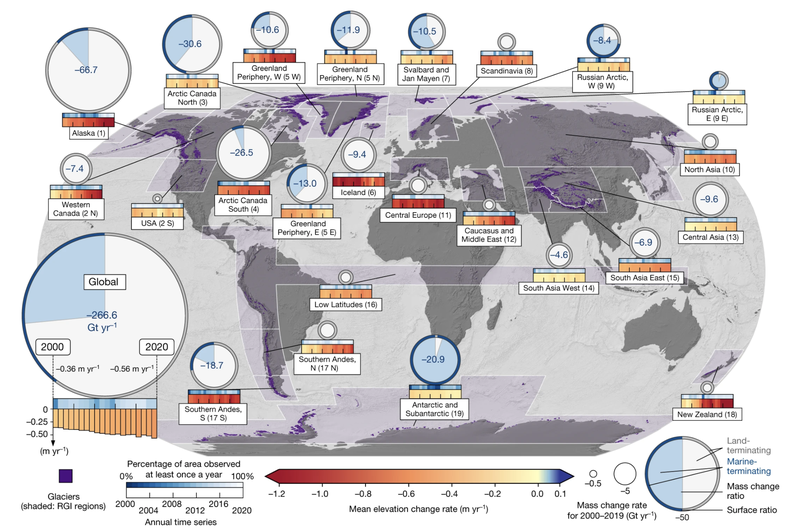
Changing patterns of evapotranspiration and precipitation and increasing temperatures, have resulted in changing patterns of soil moisture and land surface aridity worldwide, with impacts on water availability, ecosystems and agriculture. The CCI soil moisture project for example shows increases and decreases in annual surface soil moisture of up to 20% or more between the late 1970s and mid-2010s.
Land cover data from the CCI is used as supporting evidence to map the current status of tropical forests, and contribute to projected change global biodiversity at increasing levels of climate change. In the marine environment meanwhile, satellite datasets from the CCI have been used to assess changing primary production of ocean phytoplankton and its anticipated phenology by 2100 under SSP1-2.6 and SSp5-8.5.
According to Marie-Fanny Racault, a report author and member of the CCI ocean colour team:
“Enhanced development and extended implementation of these tools, especially in the most vulnerable and highly affected regions, will be paramount to support timely adaption actions to reduce climate risks under global warming.”
Studies and datasets cited in IPCC Working Group II report
Fire_cci
- Ramo, R. et al., 2021: African burned area and fire carbon emissions are strongly impacted by small fires undetected by coarse resolution satellite data. Proceedings of the National Academy of Sciences of the United States of America, 118(9), 7, doi:10.1073/pnas.2011160118
- Lizundia-Loiola, J., M. L. Pettinari and E. Chuvieco, 2020: Temporal Anomalies in Burned Area Trends: Satellite Estimations of the Amazonian 2019 Fire Crisis. Remote Sensing, 12(1), doi:10.3390/rs12010151.
- Forkel, M. et al., 2019: Recent global and regional trends in burned area and their compensating environmental controls. Environmental Research Communications, 1(5), 051005, doi:10.1088/2515-7620/ab25d2.
Glaciers_cci
- Brun, F. et al., 2017: A spatially resolved estimate of High Mountain Asia glacier mass balances from 2000 to 2016. Nature Geoscience, 10, 668, doi:10.1038/ngeo2999
- Hugonnet, R. et al., 2021: Accelerated global glacier mass loss in the early twenty-first century. Nature, 592(7856), 726-731, doi:10.1038/s41586-021-03436-z
- Shugar, D. H. et al., 2021: A massive rock and ice avalanche caused the 2021 disaster at Chamoli, Indian Himalaya. Science, 373(6552), 300-306, doi:10.1126/science.abh4455
- Zemp, M. et al., 2019: Global glacier mass changes and their contributions to sea-level rise from 1961 to 2016. Nature, 31 568 (7752), 382-386, doi:10.1038/s41586-019-1071-0
- Zemp, M. et al., 2015: Historically unprecedented global glacier decline in the early 21st century. Journal of glaciology, 61(228), 745-762p et al., 2015
Lakes_cci
- Woolway, R. I. et al., 2020b: Global lake responses to climate change. Nature Reviews Earth & Environment, 1(8), 46 388-403, doi:10.1038/s43017-020-0067-5.
- Woolway, R. I., E. Jennings and L. Carrea, 2020a: Impact of the 2018 European heatwave on lake surface water temperature. Inland Waters, 10(3), 322-332, doi:10.1080/20442041.2020.1712180.
- Woolway, R. I. et al., 2021: Lake heatwaves under climate change. Nature, 589(7842), 402-407. doi: 10.1038/s41586-020-03119-1
- Woolway, R. I. and S. C. Maberly, 2020: Climate velocity in inland standing waters. Nat. Clim. Change, 10(12), 1124-1129, doi:10.1038/s41558-020-0889-7
- Woolway, R. I. et al., 2021: Phenological shifts in lake stratification under climate change. Nature Communications, 12(1), 2318, doi:10.1038/s41467-021-22657-4
- Bruce, L. C. et al., 2018: A multi-lake comparative analysis of the General Lake Model (GLM): Stress-testing across a global observatory network. Environmental Modelling & Software, 102, 274-291, doi:10.1016/j.envsoft.2017.11.016
- Jennings, E. et al., 2021: Ecological Consequences of Climate Extremes for Lakes. Earth Systems and Environmental Sciences.
- Kraemer, B.M., Pilla, R.M., Woolway, R.I. et al. Climate change drives widespread shifts in lake thermal habitat. Nat. Clim. Chang. 11, 521–529 (2021). https://doi.org/10.1038/s41558-021-01060-3
- Stockwell, J. D. et al., 2020: Storm impacts on phytoplankton community dynamics in lakes. Global Change Biology,26(5), 2756-2784, doi:10.1111/gcb.15033
- Jane, S. F. et al., 2021: Widespread deoxygenation of temperate lakes. Nature, 594(7861), 66-70. https://doi.org/10.1038/s41586-021-03550-y
Land Cover_cci
- European Space Agency Climate Change Initiative Land Cover dataset (ESA 2017).
- ESA, 2018: Land Cover CCI Climate Research Data Package (CRDP), European Space Agency. Available at: http://www.esa-landcover-cci.org/?q=node/164 (accessed 07/06/2021) (accessed 07/06/2021).
Snow_cci
- Mudryk, L. R. et al., 2018: Canadian snow and sea ice: historical trends and projections. The Cryosphere, 12(4), 1157-1176, doi:10.5194/tc-12-1157-2018.
- Mudryk, L.R., Dawson, J., Howell, S.E.L. et al. Impact of 1, 2 and 4 °C of global warming on ship navigation in the Canadian Arctic. Nat. Clim. Chang. 11, 673–679 (2021). https://doi.org/10.1038/s41558-021-01087-6
- Pulliainen, J. et al., 2020: Patterns and trends of Northern Hemisphere snow mass from 1980 to 2018. Nature, 581, (7808), 294-298, doi:10.1038/s41586-020-2258-0
- Mudryk, L. et al., 2020: Historical Northern Hemisphere snow cover trends and projected changes in the CMIP6 multi model ensemble. The Cryosphere, 14 (7), 2495-2514.
- Mudryk, L. R., P. J. Kushner, C. Derksen and C. Thackeray, 2017: Snow cover response to temperature in observational and climate model ensembles. Geophysical Research Letters, 44 (2), 919-926, doi:10.1002/2016gl071789
- Thackeray, C. W., C. Derksen, C. G. Fletcher and A. Hall, 2019: Snow and Climate: Feedbacks, Drivers, and Indices of Change. Current Climate Change Reports, 5 (4), 322-333, doi:10.1007/s40641-019-00143-w
Ocean Colour_cci
- Racault, M.-F. et al., 2019: Environmental Reservoirs of Vibrio cholerae: Challenges and Opportunities for Ocean-Color Remote Sensing. Remote Sensing, 11(23), doi:10.3390/rs11232763
- Kulk, G. et al., 2020: Primary Production, an Index of Climate Change in the Ocean: Satellite-Based Estimates over Two Decades. Remote Sensing, 12(5), doi:10.3390/rs12050826
- Racault, M.-F., S. Sathyendranath, N. Menon and T. Platt, 2017: Phenological responses to ENSO in the global oceans. Surveys in Geophysics, 38(1), 277-293, doi:10.1007/s10712-016-9391-1
- Campbell, A. M., M.-F. Racault, S. Goult and A. Laurenson, 2020: Cholera Risk: A Machine Learning Approach Applied to Essential Climate Variables. International Journal of Environmental Research and Public Health, 17(24), doi:10.3390/ijerph17249378
Permafrost_cci
- Forbes BC et al. 2016 Sea ice, rain-on-snow and tundra reindeer nomadism in Arctic Russia. Biol. Lett. 12: 20160466. http://dx.doi.org/10.1098/rsbl.2016.0466
Sea level
- Cazenave, A. et al., 2018: Global sea-level budget 1993-present. Earth System Science Data, 10(3), 1551-1590, doi:10.5194/essd-10-1551-2018
Soil Moisture_cci
- van der Schalie, R. et al., 2021: ESA Climate Change Initiative Plus Soil Moisture Product User Guide (PUG) 44 Supporting Product Version v06.1. E