22 de febrero de 2021
Remote sensing of tipping points in the climate system
A forum held online last month (26-29 January 2021) highlighted research opportunities
A forum held online last month (26-29 January 2021) brought modellers and the remote-sensing community together to discuss how Earth observations can contribute to our understanding of tipping elements in the climate system and help with early warning of change.
Hosted by the International Space Science Institute and convened by ESA Climate Office and the Future Earth AIMES project, the interdisciplinary meeting highlighted research opportunities, challenges, and recommendations that will be published in two commentary papers.
The thematic areas explored during breakout sessions were tipping elements in the cryosphere - the planet’s snow and ice-covered regions; the terrestrial biosphere; and the atmosphere and ocean and its biota.
Climate tipping elements and risk
Many aspects of the climate are sensitive to small disrupting changes that could trigger an abrupt change in the system into a new stable state. Even at relatively low levels of global warming, systems that exhibit these instabilities could accelerate global warming through climate feedbacks or cause other cascading impacts. These ‘tipping elements’, or ‘large-scale discontinuities in the climate system’, as UNFCCC IPCC reports refer to them, have been assigned successively greater risk with each IPCC report since 2001.
With 2 degrees of warming - relative to pre-industrial levels - there is a high risk of large-scale die-off of coral reefs; many warm water corals have already crossed the tipping point for bleaching. Disappearance of alpine glaciers, loss of the Greenland ice sheet and the West Antarctic Ice Sheet, and an absence of Arctic summer sea ice are also anticipated.
At our current warming level of 1.1 degrees, we are already seeing change in the climate system accelerate, with potentially serious knock-on effects. For example, the substantial increase in ice lost from the Greenland ice sheet over the past two decades puts it on track this century to be the greatest loss in 12,000 years. As well as contributing to sea level rise, the meltwater released by the Greenland ice sheet could be affecting the overturning circulation of water in the Atlantic ocean, which has slowed by 15% since the 1950s. The Atlantic overturning circulation is important for transporting heat from the tropics to higher latitudes; significant reductions in it would impact agricultural and ecosystem productivity across the planet.
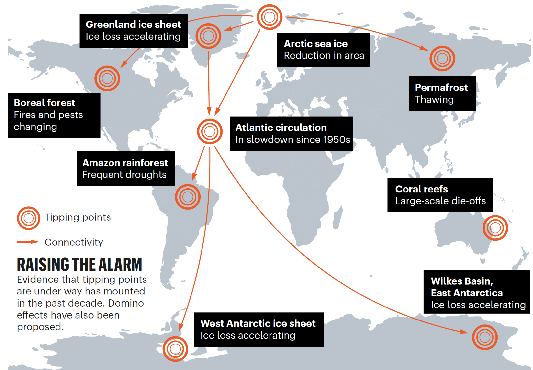
Figure 1. Climate tipping elements that could cross this century due to human activities (from Lenton et. al., 2019, Nature)
Day 1 of the forum focused on the current state-of-the-art for observing tipping elements across the different spheres, with overview presentations from science leaders and leaders for the breakout groups - Tim Lenton, Ricarda Winkelmann, Victor Brovkin, Alessandra Conversi and Didier Swingedouw. The breakouts used a risk framework (Lenton et al., 2019) to identify tipping elements and their internal feedbacks, what drives them, timescales for intervening and over which they occur, the severity of their impacts on society, and indicators for monitoring them. Tipping elements within our current time horizon up to the end of the current century were prioritised due to their relevance to people and policymakers.
There was reflection on both the political and geographical scale of an impact – for example lake eutrophication is damaging to the local ecology and human populations that depend on the lake resource for food and income, but has little widescale impact. Participants also discussed broader priorities for understanding ‘significant change’ in the climate system.
Tipping elements in oceans
Observational evidence for the tipping of the Atlantic ocean circulation subpolar gyre over the past century is consistent with model projections of tipping within ten years (Swingedouw et al, 2020). From space, observations of sea level, sea surface salinity and measurements of the strength of gravity are important for monitoring ocean current stability (Estella-Perez et al., 2020). Vertical profiles of atmospheric gas concentrations have been used to calibrate a global climate model for a study examining switches in cloud feedbacks over the Southern Ocean, with important consequences for the Earth’s climate sensitivity to levels of carbon dioxide in the atmosphere (Bjordal et al., 2020).
Observations of marine life over recent decades have shown that abrupt regime shifts are common, on the rise (Beaugrand et al., 2019) and coincide with changes in climate and hence water temperature. Circulation changes, extreme events and ocean acidification are also driving change. Coral ecosystems are becoming algal in the tropics, and algal ecosystems are becoming barrens in temperate regions. The Arctic is anticipated to experience the most dramatic shifts in the coming years with the continued opening up of sea ice in response to temperature rise driving significant ecosystem change.
Tipping elements in the biosphere
Over land, the use of a space-for-time substitution method for identifying candidate tipping elements was highlighted with illustrative examples such as shifts from tropical forest to savanna and the stability of these alternate states under the same mean annual precipitation, subject to human impact effects (Hirota et al., 2011, Wuyts et al., 2017). There are also different states of stability for northern boreal forest depending on species’ resilience (Abis & Brovkin, 2019). Arctic greening investigations using datasets of leaf area index alongside Earth System Models have found an emergent constraint on the increase in photosynthetic carbon fixation, referred to as Gross Primary Production (Winkler et al., 2019); greening of drylands can be studied using land use data; and the impact of abrupt permafrost thaw needs analysis because it is likely to be a significant source of carbon emissions over the coming decades (Turetsky et al., 2020), making use of observations of wetlands, lakes, vegetation, active layer, ground ice, snow and fire products.
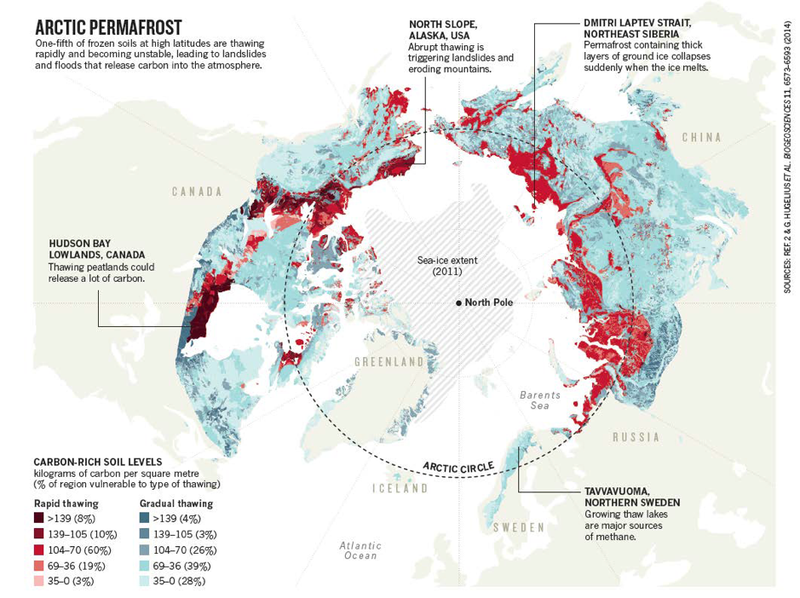
Figure 2. Map of thawing Arctic permafrost (from Turetsky et al., Nature, 2019)
Tipping elements in the cryosphere
Some of the most high profile and drastic changes across the planet are taking place in the cryosphere, with glacier calving and the breakup of marine ice shelves widely reported. Satellite data are essential for monitoring the rapid changes affecting the Earth’s snow and ice-covered environments. More and more surface melting has been observed over the Greenland ice sheet, with extreme melt events observed in 2010, 2012 and 2019. This ice sheet could have a tipping point towards complete loss at a global warming threshold of just 0.8 and 2.2 degrees above pre-industrial temperatures, mainly due to the positive feedback between surface elevation and air temperature (Levermann et al., 2013), contributing about 3 metres of sea-level rise in the coming centuries to millennia.
On the West coast of Antarctica, accelerating retreat of the Ice Sheet’s grounding line suggests that a tipping point has already been crossed in the Amundsen Sea area, driven by rising ocean temperatures that are thinning the floating ice shelves and reducing their ability to act as a buttress against ice flowing down from the ice sheet inland (Feldmann and Levermann, 2015). Tipping points for the Ice Sheet as a whole could exist at around 2 degrees and 6 and 9 degrees above pre-industrial temperatures, causing ice loss that would not be regained even if temperatures are reversed to present-day levels (Garbe, et al., 2020).
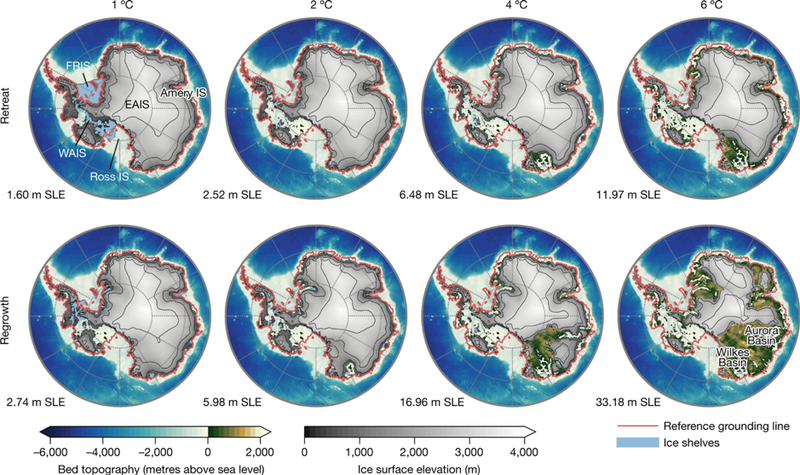
Figure 3. The equilibrium ice-sheet surface elevation is shown in metres for different warming levels (1 °C, 2 °C, 4 °C and 6 °C GMT anomaly above pre-industrial level), comparing the retreat (upper panels) and regrowth (lower panels) branch of the hysteresis curve. Ice surface-height contours are delineated at 1,000-m intervals. Grounding-line locations of the reference state are shown in red; ice shelves are marked in light blue. The absolute sea-level relevant ice-volume anomaly compared to the reference state (in m SLE), that is, the committed sea-level rise, is given for each panel. Blue shadings illustrate the bedrock depth in metres below the present-day sea level; brown shadings illustrate the bedrock elevation in metres above the present-day sea level. EAIS, East Antarctic Ice Sheet; WAIS, West Antarctic Ice Sheet (from Garbe et al., 2020).
Policy and communication needs
On day 2 the forum considered policy needs for information about climate risk from tipping points, and the communication challenges between the research and policy communities. To seed discussions, perspectives were given by author Roland Kupers of the University of Amsterdam, The Netherlands, and Liviu Stirbat of the EC DG Climate-Adaptation to Climate Change Unit.
The discussions highlighted the need to relate to people’s personal experience when talking about climate risk, using examples of nonlinear behaviour that can crop up in personal life, as opposed to the linear thinking that often governs public discourse. Also, the importance of relating climate impacts to an audience’s locality and experience, including economic impacts where possible was raised. Participants highlighted that researchers should seek to offer solutions to problems, with options, rather than problems alone.
Covid-19 and the swift international response were seen as a reason for optimism: that society can react quickly when a problem is prioritised. Examples drawn from research into social tipping points and their self-amplifying and cascading impacts also raise hopes for fast transitions towards low-carbon futures – for example sudden swings in energy and transportation markets towards investment in renewables.
Participants considered current communication challenges: that there are climate impacts we do not yet see due to delays in the climate system, and how to communicate the message that additional forcing of a system – such as release of more greenhouse gases – come with additional risk, even if a tipping point has already been crossed.
Techniques for detection
The focus for day 3 of the forum spanned techniques for improving the detection and quantification of tipping elements using remote sensing data. For example, proximity to a tipping point may be indicated in remote sensing data by characteristic statistical changes. Early warning indicators can be developed using an increasing trend in the lag-1 autocorrelation when it is correlated with an increase in variance (Boulton and Lenton, 2015). Speaker Niklas Boers of the Potsdam Institute for Climate Impact Research highlighted recent work using these characteristic statistical changes to identify the reduction in a system’s resilience, and has developed early warning indicators for Arctic sea-ice extent, Greenland ice sheet, Atlantic Meridional Overturning Circulation, the Amazon rainforest and the South American Monsoon system. The technique has also been applied to aquatic ecosystems and marine anoxic events (Gsell et al., 2016; Hennekam et al., 2020).
Other techniques highlighted by speakers Sebastian Bathiany (Wageningen University), Jonathan Donges (Potsdam Institute for Climate Impact Research) and Peter Cox (University of Exeter), included the automatic detection of extreme events and abrupt shifts in climate datasets using edge detection algorithms (Bathiany, Hidding and Scheffer, 2020); use of multiple Earth System models with climate observations to constrain a system’s sensitivity in relation to abrupt change (Eyring et al, 2019); and the use of remote sensing in a causal effect network approach to analyse interactions and drivers of tipping elements, making use of the data cube Earth System Data Lab (Runge et al., 2015; Mahecha et al., Earth System Dynamics, 2020).
Research priorities and next steps
In the final sessions of the forum participants discussed research opportunities across ocean, cryosphere and land domains, and priorities for improving our understanding of tipping elements and their early warning using remote sensing. Participants favoured a generic definition of tipping elements to include non-linearities and abrupt change detection, and considered extending the scope of risk to include extinctions and migrations more widely. Tipping element interactions and cascades, and their feasibility of study, were identified as categories for prioritising research.
The spatial resolution of satellite datasets, as well as the resolution across the frequency spectrum, are both improving, with missions in the pipeline set to bring new measurements, higher accuracy and shorter revisit times. However, these improvements have associated challenges, such as the need to bridge the scale differences between observations and models.
Participants noted the importance of methodologies to take advantage of the high resolution imagery available in remote sensing datasets to study spatial patterning and nonlinear disturbance effects – such as for monitoring forest fragmentation, Arctic thaw lake extent, or the surface melt ponds of ice shelves. They also noted new products being generated for monitoring fragile, liminal systems – such as the Global Mangrove Watch project. Monitoring of coastal and Arctic ecosystems was noted as an area needing more development.
Feedback from the meeting was very positive. In particular, modellers reported that remote sensing was viewed as an emerging opportunity, and felt that holding the meeting online meant the group was more mixed and therefore more valuable for learning new information and making new contacts. There is strong enthusiasm for follow-up collaborations and for writing papers using a collaborative platform. Participants suggested holding regular catch-up meetings, for example through the AIMES working group, to intensify the exchange between remote sensing experts and modellers as well as with stakeholders working on policy communication. The group hope to convene a session at EGU or a follow-up with an ISSI workshop building on the same topic.
References
Abis, B, Brovkin, V. Alternative tree‐cover states of the boreal ecosystem: A conceptual model. Global Ecol Biogeogr. 2019; 28: 612– 627. https://doi.org/10.1111/geb.12880
Bathiany, S., Hidding, J., and Scheffer, M., Edge Detection Reveals Abrupt and Extreme Climate Events (2020) doi: https://doi.org/10.1175/JCLI-D-19-0449.16399–6421
Beaugrand, G., Conversi, A., Atkinson, A. et al. Prediction of unprecedented biological shifts in the global ocean. Nat. Clim. Chang.9, 237–243 (2019). https://doi.org/10.1038/s41558-019-0420-1
Boulton., C., and Lenton, T., Slowing down of North Pacific climate variability, PNAS (2015) 112(37): 11496-11501. DOI:10.1073/pnas.1501781112
Bjordal, J., Storelvmo, T., Alterskjær, K. et al. Equilibrium climate sensitivity above 5 °C plausible due to state-dependent cloud feedback. Nat. Geosci. 13, 718–721 (2020). https://doi.org/10.1038/s41561-020-00649-1
Estella-Perez, V., Mignot, J., Guilyardi, E. et al. Advances in reconstructing the AMOC using sea surface observations of salinity. Clim Dyn 55, 975–992 (2020). https://doi.org/10.1007/s00382-020-05304-4
Eyring, V., Cox, P.M., Flato, G.M. et al. Taking climate model evaluation to the next level.Nature Clim Change 9, 102–110 (2019). https://doi.org/10.1038/s41558-018-0355-y
J. Feldmann, A. Levermann, Collapse of the West Antarctic Ice Sheet PNAS, Nov 2015, 112 (46) 14191-14196; DOI:10.1073/pnas.1512482112 https://www.pnas.org/content/112/46/14191
Garbe, J., Albrecht, T., Levermann, A. et al. The hysteresis of the Antarctic Ice Sheet.Nature 585, 538–544 (2020). https://doi.org/10.1038/s41586-020-2727-5
Gsell, A.S., Scharfenberger, U., Deniz Özkundakci, Annika Walters, Lars-Anders Hansson, Annette B. G. Janssen, Peeter Nõges, Philip C. Reid, Daniel E. Schindler, Ellen Van Donk, Vasilis Dakos, Rita Adrian. Early-warning indicators in empirical time series. Proceedings of the National Academy of Sciences (2016), 113 (50) E8089-E8095; DOI:10.1073/pnas.1608242113
Hennekam, R., van der Bolt, B., van Nes, E. H., de Lange, G. J., Scheffer, M., & Reichart, G.‐J. (2020). Early‐warning signals for marine anoxic events. Geophysical Research Letters, 47, e2020GL089183. https://doi.org/10.1029/2020GL089183
Lenton TM, Rockström J, Gaffney O, Rahmstorf S, Richardson K, Steffen W, Schellnhuber HJ. Climate tipping points - too risky to bet against. Nature. 2019 Nov;575(7784):592-595. doi: 10.1038/d41586-019-03595-0. PMID: 31776487.
Levermann, A., Clark, P. U., Marzeion, B., Milne, G.A., Pollard, D., Radic, V., Robinson, A., The multimillennial sea-level commitment of global warming, Proceedings of the National Academy of Sciences Aug 2013, 110 (34) 13745-13750; DOI:10.1073/pnas.1219414110
Mahecha, M. D., Gans, F., Brandt, G., Christiansen, R., Cornell, S. E., Fomferra, N., Kraemer, G., Peters, J., Bodesheim, P., Camps-Valls, G., Donges, J. F., Dorigo, W., Estupinan-Suarez, L. M., Gutierrez-Velez, V. H., Gutwin, M., Jung, M., Londoño, M. C., Miralles, D. G., Papastefanou, P., and Reichstein, M.: Earth system data cubes unravel global multivariate dynamics, Earth Syst. Dynam., 11, 201–234, https://doi.org/10.5194/esd-11-201-2020, 2020.
Runge, J., Petoukhov, V., Donges, J. et al. Identifying causal gateways and mediators in complex spatio-temporal systems. Nat Commun 6, 8502 (2015). https://doi.org/10.1038/ncomms9502
Swingedouw, D., Ifejika Speranza, C., Bartsch, A. et al. Early Warning from Space for a Few Key Tipping Points in Physical, Biological, and Social-Ecological Systems. Surv Geophys 41, 1237–1284 (2020). https://doi.org/10.1007/s10712-020-09604-6
Wuyts, B., Champneys, A. & House, J. Amazonian forest-savanna bistability and human impact. Nat Commun 8, 15519 (2017). https://doi.org/10.1038/ncomms15519
Hirota M, Holmgren M, Van Nes EH, Scheffer M. Global resilience of tropical forest and savanna to critical transitions. Science. 2011 Oct 14;334(6053):232-5. doi: 10.1126/science.1210657. PMID: 21998390.
Turetsky, M.R., et al., Nature 569, 32-34 (2019) doi: https://doi.org/10.1038/d41586-019-01313-4
Turetsky, M.R., Abbott, B.W., Jones, M.C. et al. Carbon release through abrupt permafrost thaw. Nat. Geosci. 13, 138–143 (2020). https://doi.org/10.1038/s41561-019-0526-0
Winkler, A.J., Myneni, R.B., Alexandrov, G.A. et al. Earth system models underestimate carbon fixation by plants in the high latitudes. Nat Commun 10, 885 (2019). https://doi.org/10.1038/s41467-019-08633-z